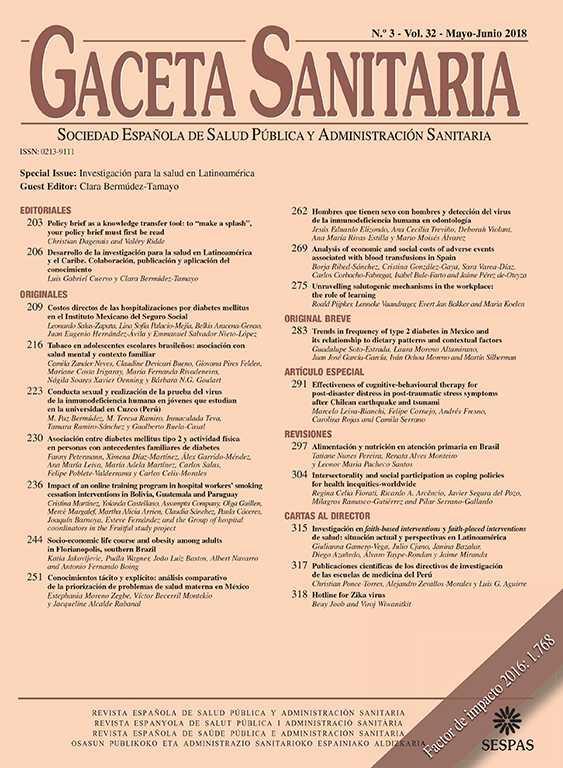
The 3rd International Nursing and Health Sciences Students and Health Care Professionals Conference (INHSP)
More infoCardiogenic shock is defined as tissue hypoperfusion due to cardiac dysfunction. It is associated with hemodynamic unstability and elevated arterial lactate as one indicator for anaerobic metabolism. Hypercatabolic state in this condition leads to increasing nutritional requirement and negative nitrogen balance. Therefore, medical nutrition therapy by considering metabolic tolerance can prevent further metabolic deterioration and loss of lean mass and improve the patient's clinical outcome.
MethodsA 44-years-old female patient with severe protein-energy malnutrition (Subjective Global Assessment Score C; MUAC 15cm) suffered from hemodynamic unstability due to cardiogenic shock and infected bronchiectasis at the infection center of Wahidin Sudirohusodo Hospital. Intake was postponed due to mean arterial pressure 56mmHg on vasopressor support and oxygen saturation below 93%. Physical examinations showed loss of subcutaneous fat, lung crackles and wheezing, muscle wasting, and pretibial edema. Laboratory assessments showed elevated arterial lactate (3.2mmol/L), hypoalbuminemia (2.4g/dL), lymphocytopenia (650/μL), elevated liver enzymes (SGOT 780U/L; SGPT 868U/L), and urine urea nitrogen (5g/24h). Nutritional therapy was started after mean arterial pressure ≥65mmHg with a stable dosage of the vasopressor drug and decreased arterial lactate level to 2.2mmol/L then given gradually with a target calorie of 1500kcal and protein 1.5–1.8g/kg ideal body weight/day using high protein diet. Arterial lactate and blood gass analyses were controlled every day to determine the target of nutritional therapy day by the day. Snakehead fish extract, zinc, vitamin B complex, Thiamine, vitamin C, vitamin A, vitamin D3, and Curcumin were supplied.
ResultAfter 15 days of nutritional therapy, the patient was discharged from the hospital with stable hemodynamic without vasopressor support, adequate nutritional intake, improvement of anthropometric parameters, and laboratory test results (arterial lactate 1.6mmol/L, albumin 3.1g/dL, lymphocyte 1.871/μL, SGOT 34U/L, SGPT 41U/L, urine urea nitrogen 0.72g/24h).
ConclusionAdequate nutritional therapy, which is planned by evaluating hemodynamic tolerance, can improve patient clinical outcomes and positive nitrogen balance in the hemodynamically unstable patient.
Cardiogenic shock is associated with hemodynamic unstable and elevated arterial lactate as one indicator for anaerobic metabolism due to low perfusion in tissue.1,2 Evaluation of hemodynamic condition can be used to determine a plan of nutritional therapy.3 Nutritional therapy consists of macronutrients and micronutrients requirements. In the critically ill patient with hypercatabolic state, nitrogen losses can reach 16–20g/day and, in some cases, up to 24g/day compared to those without catabolic stress which generally loses only 10–12g of nitrogen/day.4 Loss of body mass around 30–40% will increase the mortality rate due to starvation or protein malnutrition.5 High protein diet and adequate micronutrients intervention can improve the outcome of the patient. Delayed nutritional therapy will worsen negative nitrogen balance, nutritional status, and hypercatabolic state. On the other hand, too early nutritional intervention can worsen anaerobic metabolism in a hemodynamically unstable patient. Therefore, the nutritional goal can be planned by evaluating hemodynamic changes to determine the time for postponing nutritional therapy or increasing the nutritional target.
MethodsThis is a case report of a patient who was treated in September 2018 at the Wahidin Sudirohusodo Hospital. This case report has fulfilled the requirement of the Ethics Committee of Hasanuddin University School of Medicine. The patient and the family were given informed consent and agreed to be reported as a case report without mentioning the name and private information.
A 44-years-old female patient with severe protein-energy malnutrition with the marasmic type (Subjective Global Assessment Score-C; MUAC 15cm) was consulted from Pulmonology Departement with hemodynamic unstability due to cardiogenic shock and infected bronchiectasis since one day ago at the infection center of Wahidin Sudirohusodo Hospital, Makassar. She had a history of low dietary intake since 3 months ago due to decreased appetite and shortness of breath. Unintentional weight loss of about 4kg was present 3 months ago. Intake was postponed due to mean arterial pressure 56mmHg on vasopressor support and oxygen saturation below 93%. Physical examinations showed loss of subcutaneous fat, lung crackles and wheezing, muscle wasting, and pretibial edema. Laboratory assessments showed elevated arterial lactate (3.2mmol/L), hypoalbuminemia (2.4g/dL), lymphocytopenia (650/μL), elevated liver enzymes (SGOT 780U/L; SGPT 868U/L), and urine urea nitrogen (UUN 5g/24h). Echocardiography showed the left and right ventricle (ejection fraction 64%) with mild diastolic dysfunction of the left ventricle. Abdominal ultrasonography showed congestive liver signs. Thoracal MSCT showed dilated bronchiectasis.
Basal metabolic rate (BMR) by Harris-Benedict Formula was 983kcal, and total energy expenditure (TEE) was 1500kcal. Nutritional therapy was started after mean arterial pressure ≥65mmHg with a stable dosage of the vasopressor drug for 6h and arterial lactate 2.2mmol/L with 30–40% of TEE on 2–3 days after stable hemodynamic. Macronutrients were given for patients with protein 0.8–1.3g/ideal body weight (IBW)/day, carbohydrates 45–50%, and lipid 30% for seven days after hemodynamic unstability onset. Protein was gradually increased to 1.5–1.8g/IBW/day using a high protein diet and high branched-chain amino acid formula to create a positive nitrogen balance based on the UUN result. The positive nitrogen balance target was +4. In addition, routine arterial lactate and blood gas analyses were assessed to control hemodynamic tolerance.
ResultAfter arterial lactate was below 2.0mmol/L with tappering-off vasopressor dosage, nutritional therapy was planned for 100% TEE on day 8 (Figs. 1 and 2). In addition, supplementations of Snakehead fish extract, zinc 20mg, vitamin B complex, thiamine 300mg, vitamin A 6000IU, vitamin C 500mg, and vitamin D3 400IU were given to improve immune and metabolic function. After 15 days of nutritional therapy, the patient was discharged from the hospital with stable hemodynamic without vasopressor support, adequate oral intake (90–95% of TEE), improvement of anthropometric parameters, and laboratory test results (arterial lactate 1.6mmol/L, albumin 3.1g/dl, lymphocyte 1.871/μL, SGOT 34U/L, SGPT 41U/L, UUN 0.72g/24h) (Figs. 3 and 4).
DiscussionThe patient was admitted to the emergency department with diagnosis of cardiogenic shock (Fig. 5). She had mean arterial pressure of 56mmHg on vasopressor support, oxygen saturation below 93%, and elevated arterial lactate level to 3.2mmol/L. Hyperlactatemia in this patient was hyperlactatemia type A that occurred due to impaired tissue perfusion.6,7 Furthermore, periodic control of arterial lactate level was carried out to assess the hemodynamic status to determine nutritional therapy target.8 Cuthberson, the first person who described the metabolic changes that occurred after major trauma in 1942, divided metabolic response to trauma into Ebb and Flow Phases.9 The Ebb phase begins within the first hour after trauma and can last up to 24–48h, characterized by a decrease in total energy requirements and a decrease in urinary nitrogen excretion. Targets in this phase are hemodynamic adaptation and stable tissue perfusion. The flow phase is also known as the catabolic phase. The metabolic response in this phase is mediated by catabolic hormones (such as glucagon, catecholamines, and corticosteroids) and accompanied by insulin resistance. Acute phase protein synthesis in liver, pro-inflammatory cytokines and free radicals are also increase in this phase.10 Hyperlactatemia in critically ill patients and particularly those in shock is generally interpreted as a marker of secondary anaerobic metabolism due to inadequate oxygen supply inducing cellular distress.2 In this case, arterial lactate test was performed 18h (3.2mmol/L) after the onset of shock, then re-assessed 24h later (2.2mmol/L), and controlled every day till lactate level 1.6mmol/L to plan 100% nutritional target. Mizuki et al. reported lactate clearance as one of the most important predictors of in-hospital mortality in patient treated with extracorporeal cardiopulmonary resuscitation after cardiac arrest.11
In the critical phase, the priority of protein synthesis in liver is to form acute-phase proteins, such as C-reactive protein, α1-acid glycoprotein, α1-protease inhibitor, fibrinogen, and haptoglobin, than constitutive proteins, such as albumin, prealbumin, and retinol-binding protein.4 Albumin level in this patient was 2.4g/dL at admission and gradually increased up to 3.1g/dL, which was correlated with the decrement of UUN from 5g/24h to 0.72g/24h (Fig. 5). Loss of lean mass about 30–40% due to negative nitrogen balance and undernourishment are correlated with high mortality rates.5,12 Data from an observational study showed that protein intake of 1.2–1.5g/kg IBW/day was associated with a better outcome. Ishibasi et al. showed that protein intake of 1.5g/kg BW/day was associated with a minimal negative nitrogen balance.13 Negative nitrogen balance (−2.89) in this patient was calculated from UUN (5g/24h) by using a nitrogen balance correction formula in critical patients (nitrogen balance=(protein intake/6.25)−(UUN/0.85)−4).5 Therefore, the protein target was increased to 1.8g/kg IBW/day to achieve positive nitrogen balance (+0.5) using high protein formula.14 After 15 days of nutritional therapy, UUN was 0.72g/24h with a higher estimated positive nitrogen balance (+4.72). Positive nitrogen balance (around +4g/day) can support protein synthesis by 25g/day and increase body mass up to 100g/day.10
Elevated liver enzymes can be caused by acute liver injury due to hepatic hypoperfusion or due to acute cardiogenic liver injury. This condition is commonly seen in a patient with decreased cardiac output, cor pulmonale disease, and acute decompensated heart failure. In acute circulation disruption, blood flow to the liver decreases about 10% for every 10mmHg decrease in arterial pressure.3 The role of Branched Chain Amino Acids (BCAAs) in acute liver injury is still controversial. There is no recommendation about it. However, several animal studies have shown the role of BCAAs in animals experiencing an acute liver injury. Kitagawa et al. reported that BCAAs play a role in improving the liver condition in mice that experience acute liver injury due to the ischemic–reperfusion phase through improved microcirculation, inflammatory response, and leukocyte adhesion.15 Daily Snakeheadfish extract, zinc 20mg, vitamin B complex, Thiamine 300mg, vitamin C 500mg, vitamin A 6000IU, vitamin D3 600IU, and Curcumin 1.200mg were given. Vitamin D has a role in modulating innate and adaptive immune responses.16 Snakehead fish extract contains high protein, albumin, and several micronutrients.17,18 This extract increases albumin levels in hypoalbuminemia patients and modulates the immune response by molecular and immunological mechanisms.19 Zinc plays a role in boosting the immune system by optimizing A20mRNA upregulation and decreasing NF-κB activation, suppressing activation of TNF-α, IL-1β, and IL-8. Zinc supplementation can reduce oxidative stress.20 Parikh et al. reported that vitamin C 5mg/kg/day in children or 200mg/day in adults could optimize mitochondrial function and reduce arterial lactate level.21In vitro studies demonstrate that T cell development requires vitamin C, while vitamin C also enhances T cell proliferation and may influence T cell function.22
ConclusionAdequate medical nutrition therapy, which is planned by evaluating hemodynamic tolerance, can improve clinical outcomes and positive nitrogen balance in hemodynamically unstable patients.
Conflicts of interestThe authors declare no conflict of interest.
Peer-review under responsibility of the scientific committee of the 3rd International Nursing, Health Science Students & Health Care Professionals Conference. Full-text and the content of it is under responsibility of authors of the article.